Rechargeable batteries with conversion type cathodes/anodes are highly sought-after due to their ability to achieve much higher capacity through multi-electron transfer reactions. Elemental sulfur (S) is one of the most attractive materials amongst all conversion-based cathodes because of its high theoretical capacity (~1675 mAh/g – 5-10-fold higher than Li-ion batteries), natural abundance, non-toxicity, and cost-effectiveness. A key interest of our group is to combine fundamental and applied research to develop next generation, largely, Lithium-sulfur batteries. Additionally, we also research ion-batteries, including Li-ion, Na-ion and also alternate anode sulfur batteries, such as Na-S, Mg-S.
I. Design of Electrode Architectures and Chemistries for lithium-sulfur batteries
A primary sulfur cathode challenge is the dissolution of intermediate redox products, namely polysulfides, in common electrolytes causing loss of active material resulting in limited cyclic stability, underutilization of sulfur, rapid self-discharge, and low coulombic efficiency in sulfur batteries. Our work in this field has focused on design of novel electrode architectures and chemistry to physically and/or chemically entrap intermediate polysulfides to slow down the kinetics of the notorious polysulfide shuttling phenomenon and eventually enhance cycle stability and reduce self-discharge. We often integrate our design work with in-operando and postmortem spectroscopy to elucidate redox mechanisms and host-polysulfide chemical interactions as discussed in more detail in the next section.
In this thrust, we work with a variety of cathode chemistries ranging from simple carbon-based host architectures that physically confine polysulfides and more sophisticated transition metal oxides/nitrides–based chemistries to trigger Lewis acid-base interactions to chemically trap the polysulfides. In another research direction, we develop new cathode chemistries/crystal structures that tailor the reaction mechanism for complete elimination of the intermediate polysulfides enabling stable capacity for thousands of charge-discharge cycles. On the anode side, we study both lithium and other cheaper, more abundant alternates such as sodium, magnesium. For device testing, we routinely assemble and test coin cells (~1 cm2) as well as prototype-level pouch cells (25 cm2) in our lab at Drexel. See facilities tab for a list of equipment.
Examples of our work include:
1) TiO phase stabilized into freestanding nanofibers as strong polysulfide immobilizer in Li–S batteries: evidence for Lewis acid–base interactions. (Reference)
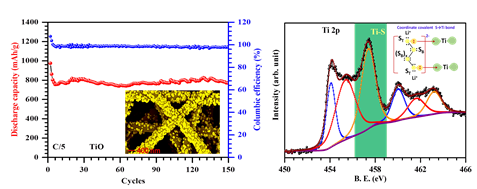
2) Tuning functional 2D MXene (Ref 1, Ref 2) nanosheets to enable efficient sulfur utilization in high loading lithium-sulfur batteries.
3) Tuning sulfur-based active material to enable solid-solid direct conversion for stable capacity for thousands of cycles.
II. In-operando and Postmortem Spectroscopy to Understand Redox Mechanisms in Battery Devices.
Although conversion-reaction-based lithium-sulfur (Li-S) battery is one of the most promising, closest-to-market technologies available due to its high theoretical energy density, however, before this technology can become a commercial reality, several fundamental challenges associated with the complex electrochemically reversible redox reactions of S8 to Li2S/Li2S2, the high solubility of intermediate polysulfide species into the electrolyte and lithium dendrite formation, among others, need to be addressed. To this end, we integrate in-operando spectroscopy (FTIR, Raman) and post-mortem (cycled cells) spectroscopy/microscopy (IR, Raman, XRD, XPS, SEM, TEM) to elucidate electrochemical behavior to guide battery materials research.
A primary focus of this thrust is to develop the ability to observe molecular-level changes in real-time during device operation under representative cell operating conditions to investigate electrochemical redox mechanisms, evolution of intermediate products, and to study host-product and product-electrolyte interactions at the electrode-electrolyte interface.
Examples of our work include:
1) Polysulfide Speciation and Electrolyte Interactions in Lithium–Sulfur Batteries with In-Situ Infrared Spectroelectrochemistry. (Reference)
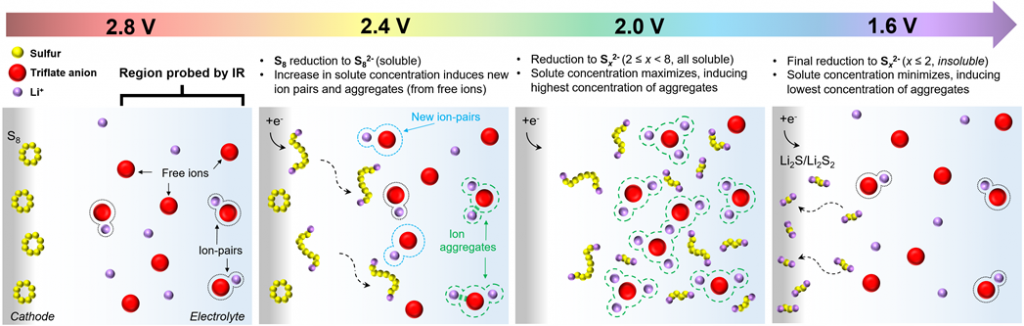
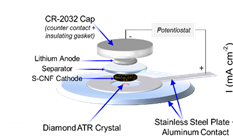
2) In situ FTIR study on organo-polysulfide evolution in CNF/sulfur copolymer cathodes for Li-S batteries: Effect of sulfur chain length on the electrochemical performance.
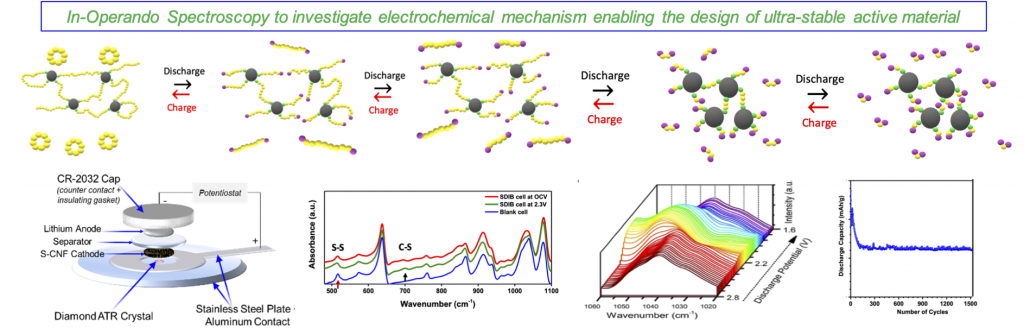
III. Designing optimal electrolyte additives for Sulfur batteries
A practical and economical approach to improve the performance of Li-S batteries, without adding excess weight and hindering their achievable energy density, is introducing appropriate electrolyte additives. These additives are used to provide protection for Li metal anode, suppress polysulfide shuttle, or mediate redox reactions happening in each cycle. The result of these studies can be expanded to alternate metal-S batteries.
In this regard, our research on electrolyte additives has been focused on developing and understanding novel additive chemistries – their redox activity, their effect on battery performance, fundamental role they play and interactions they exhibit with reaction products, and mediation mechanisms.
Examples of our work include:
1) Revisiting the use of electrolyte additives in Li–S batteries: the role of porosity of sulfur host materials. (Reference)
2) Thiourea as a “dual-role” electrolyte additive for simultaneous polysulfide shuttle inhibition and redox mediation for enhanced reaction kinetics
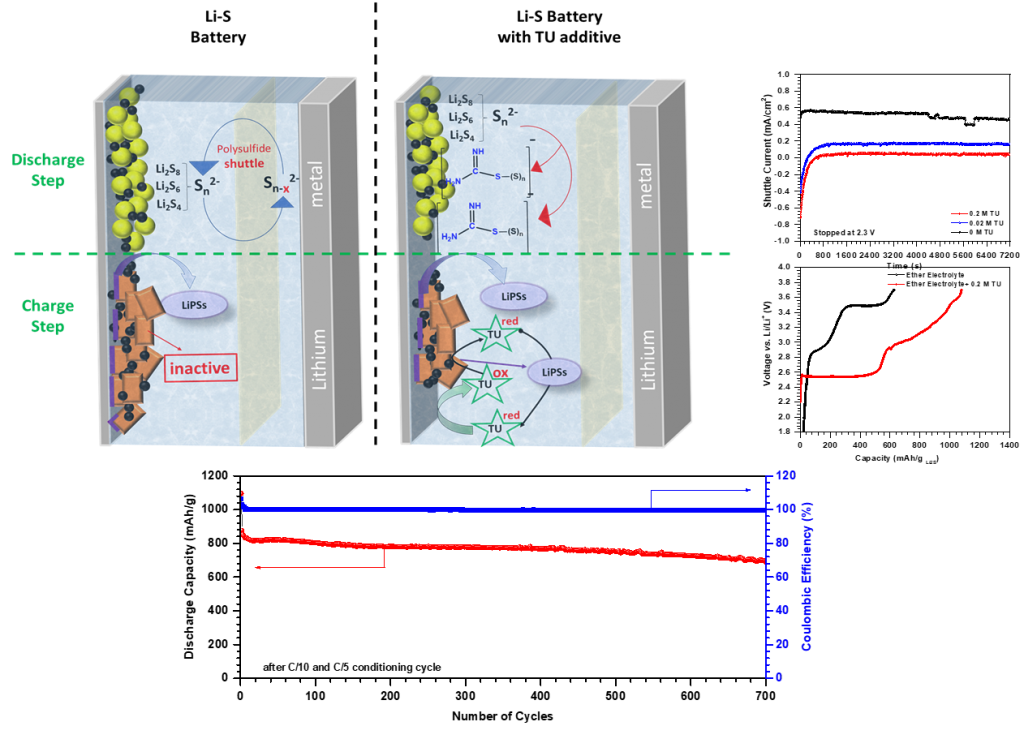