As scientists, we like to solve puzzles about the natural world. But as engineers, we are drawn to problems with great societal and economic importance. The Tang Lab combines those two roles by studying the fundamental physics and chemistry of systems and materials for electrochemical energy storage and conversion.
The best example of an electrochemical energy storage system is a battery, such as those used in laptops, cell phones, and, increasingly, cars. This kind of device stores electricity in the form of chemical bonds, then reverses those bonds when the electricity is needed in a different time or place than it is generated. An electrochemical energy conversion system converts electricity into valuable chemicals or vice versa. For example, most of the world’s aluminum is produced by reducing molten salts in electrochemical cells. As electricity from wind and solar (but also methane) gets cheaper and cheaper, there is more and more interest in driving traditional chemical engineering processes with electricity instead of heat.
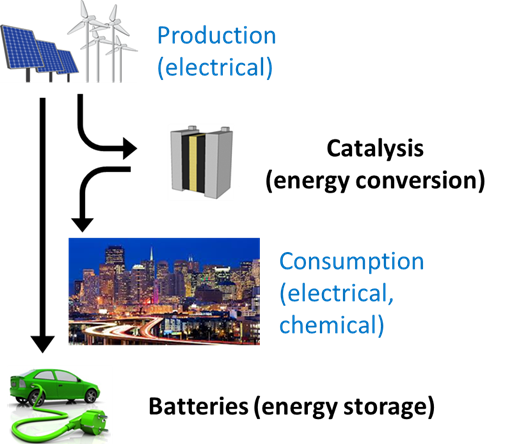
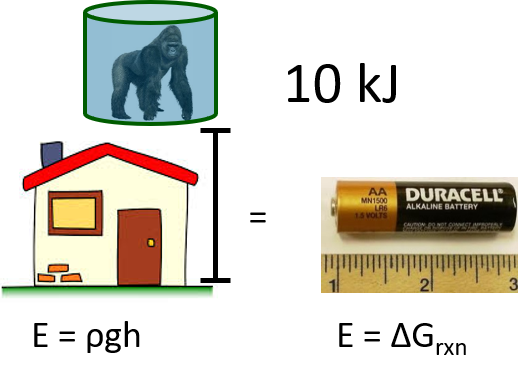
Electrochemical systems are one of the most promising routes for scalable electricity storage. Long-term storage is the Achilles’ heel of intermittent renewables like wind and solar because electrical energy must be converted to thermal, mechanical, or chemical energy. No current technology can accomplish this task at scale. The diversity of atomic arrangements in molecules makes chemical energy by far the most versatile. More importantly, the energy stored in chemical bonds dwarfs thermal or mechanical methods. 10 kJ can be stored mechanically, by pumping 100 gallons of water ten feet uphill (minus conversion efficiency), or chemically, by charging a single AA battery.
As one might imagine, electrochemical systems have some pretty complicated physics. There are lots of densely packed molecules moving around, many of them charged. There are at least two (and usually more) reactions at interfaces, and often reactions in solution as well. Our group thrives on this complexity because we excel at mechanistic analyses. What this means is that we think very carefully about a complicated system with many moving parts, distill the confusion down to a straightforward question, then come up with a clever method to answer the question. Often, that method involves comparing a physics-based model to some experimental measurements of current and voltage. Here are a few examples of the kinds of questions we ask (and answer):
- Is hydrogen electrocatalysis slower in acid than in base because the mechanism changes? Answer: no.
- Which is more important to Li-ion battery rate capability, ion or electron transport? Answer: electrons.
- Do the protective layers that form on nonaqueous battery electrodes fail because of transport (morphology) or kinetics (electronic structure)? Answer: kinetics.
Sometimes, our answer to the fundamental question leads us to propose a new material or device that can solve a problem. We work with materials scientists, polymer chemists and other collaborators to test these ideas. We can and do make our own materials, but have generally found that working with other people yields better results and is more fun. If you think you have a problem that we could help with, send Maureen an email.